Spotlight on Research #6: Baby Galaxies
Research Highlight | November 01, 2013
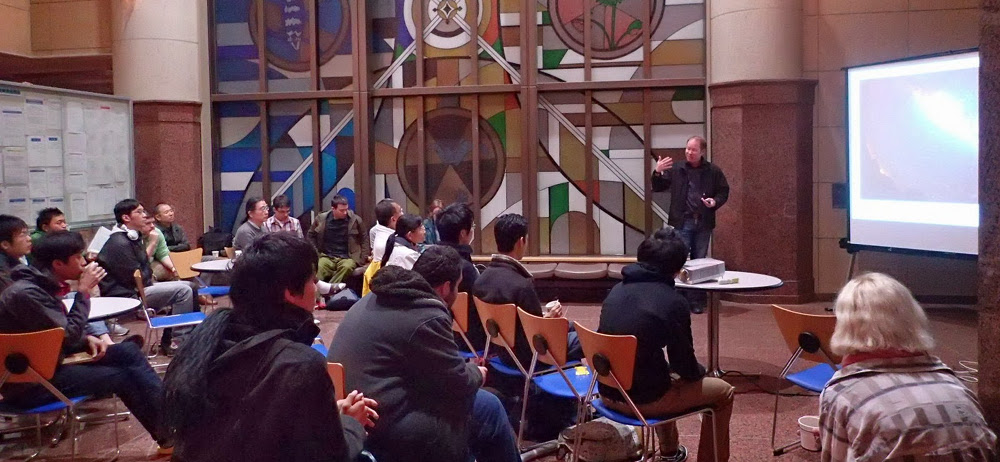
Hold an American dime (a coin slightly smaller than a 1 yen piece) at arms length and you can just make out the eye of Franklin D. Roosevelt, the 32nd President of the USA whose profile is etched on the silver surface. Lift the eye on that coin up to the sky and you’re looking at a region containing over 10,000 galaxies.
In 1995, the Hubble Space Telescope was trained on a small patch of space in the constellation Ursa Major. So tiny was this region that astronomers could only make out a few stars belonging to our Milky Way. To all intents and purposes, the space telescope was pointing at nothing at all.
After 10 days, scientists examined the results. Instead of a pool of empty blackness, they saw the light of 3,000 galaxies packed into a minute one 24-millionth of the entire sky. In 2003, the observation was repeated for an equally tiny region of space in the constellation Fornax. The result was over 10,000 galaxies. These images are known as the ‘Hubble Deep Field’ and ‘Hubble Ultra Deep Field’ and became some of the most famous results in the space telescope’s legacy.
For their second public Sci-Tech talk, the Faculty of Science’s Office of International Academic Support invited Professor John Wise from the Georgia Institute of Technology in the USA to talk about his search to understand the formation of the very first galaxies in the Universe.
John explained that when you look at the Hubble Deep Field, you do not see the galaxies as they are now. Rather, you are viewing galaxies born throughout the Universe’s life, stretching back billions of years. The reason for this strange time alignment is that light travels at a fixed, finite speed. This means that we see an object not as it is now, but how it was at the moment when light left its surface.
Since light travels 300,000 km every second, this delay in light leaving an object and reaching your eyes is infinitesimally small for daily encounters. For light to travel to you from a corner shop 100 meters away, takes a tiny 0.0000003 seconds. However, step into the vastness of space and this wait becomes incredibly important.
Light travelling to us from the moon takes 0.3 seconds. From the Sun, 8 minutes. For light to reach us from the Andromeda Galaxy, we have to wait 2.4 million years. The Andromeda we see is therefore the galaxy as it was 2.4 million years ago, before humans ever evolved on Earth.
At a mere 24,000,000,000,000,000,000 km away, Andromeda is one of our nearest galactic neighbours. Look further afield, and we start to view galaxies that are living in a much younger universe; A universe that has only just began to build galaxies.
“At the start of the Universe,” Wise explains. “There were no stars or galaxies, only a mass of electrons, protons and particles of light. But when it was only 380,000 years old, the Universe had cooled enough to allow the electrons and protons to join and make atoms.”
This point in the Universe’s 13.8 billion year history is known as ‘Recombination’; a descriptive name to mark electrons and protons combining to make the lightest elements: Hydrogen, Helium and a sprinkling of Lithium.
Then, the Universe goes dark.
For the next 100 million years, the Universe consists of neutral atoms that produce no light nor scatter the current populations of light particles. Astrophysicists, who rely on these light scatterings to see, refer to this time as the ‘Dark Ages’ where they are blind to the Universe’s evolution.
Then, something big happens.
An event big enough that it sweeps across the Universe, separating electrons from their atom’s nucleus in an event called ‘Reionisation’.
“It was like a phase transition,” Wise describes. “Similar to when you go from ice to water. Except the Universe went from neutral to ionised and we were now able to see it was full of young galaxies.”
But what caused this massive Universe phase transition? Wise suspected that the first galaxies were the cause, but with nothing visible during the Dark Ages, how is this proved?
To tackle this problem, Wise turned to computer models to map the Universe’s evolution through its invisible period. Before he could model the formation of the first galaxy, however, Wise had to first ask when the first stars appeared.
“Before any galaxies formed, the first stars had to be created,” Wise elaborates. “These were individual stars, forming alone rather than in galaxies as they do today.”
As the pool of electrons and protons join at ‘Recombination’ to create atoms, small differences in density were already in place from the first fraction of a second after the Big Bang. The regions of slightly higher density began to exert a stronger gravitational pull on the atoms, drawing the gas towards them. Eventually, these clouds of gas grew massive enough to begin to collapse and star was born in their centre.
Their isolated location wasn’t the only thing that made the first stars different from our own Sun. With only the light elements of hydrogen, helium and lithium from which to form, these stars lacked the heavier atoms astrophysicists refer to as ‘metals’. Due to their more complicated atomic structure, metals are excellent at cooling gas. Without them, the gas cloud was unable to collapse as effectively, producing very massive stars with short lifetimes.
“We don’t see any of these stars in our galaxy today because their lifetimes were so short,” Wise points out. “Our Sun will live for about 10 billion years, but a star 8 times heavier would only live for 20 million years; thousands of times shorter. That’s a huge difference! The first star might be ten times as massive with an even shorter lifetime.”
In the heart of the newly formed star, a key process then began: that of creating the heavier elements. Compressed by gravity, hydrogen and helium fuse to form carbon, nitrogen and oxygen in a process known as ‘stellar nucleosynthesis’. As it reaches the end of its short life, the first stars explode in an event called a ‘supernovae’, spewing the heavier metals cooked within their centre out into the Universe.
The dense regions of space that have formed the first stars now begin to merge themselves. Multiple pools of gas come together to produce a larger reservoir from which the first galaxies begin to take shape.
Consisting of dense, cold gas, filled with metals from the first stars, these first galaxies turn star formation into a business. More stars form, now in much closer vicinity, and the combined heat they produce radiates out through the galaxy. This new source of energy hits the neutral atoms and strips their electrons, ionising the gas.
As the fields of ionised gas surrounding the first galaxies expand, they overlap and merge, resulting in the whole Universe becoming ionised. Wise’s simulations show this in action, with pockets of ionised gas and metals spreading to fill all of space.
While this uncovers the source of the Universe’s ionisation, the impact of the first galaxies does not stop there. In the same way that the regions of first star formation had combined to form galaxies, further mergers pull the galactic siblings together. The result is the birth of a galaxy like our own Milky Way.
These first galaxies, Wise concludes, therefore form a single but important piece of the puzzle that ultimately leads to our own existence here in the Milky Way.
Author: Elizabeth Tasker
Look out for further details and events on the OAIS Faculty of Science FaceBook page.