200-year-old geology puzzle resolved
Research Press Release | November 24, 2023
Joint press release by the University of Michigan and Hokkaido University.
To build mountains from dolomite, a common mineral, it must periodically dissolve. This counter-intuitive lesson could help make new defect-free semiconductors and more.
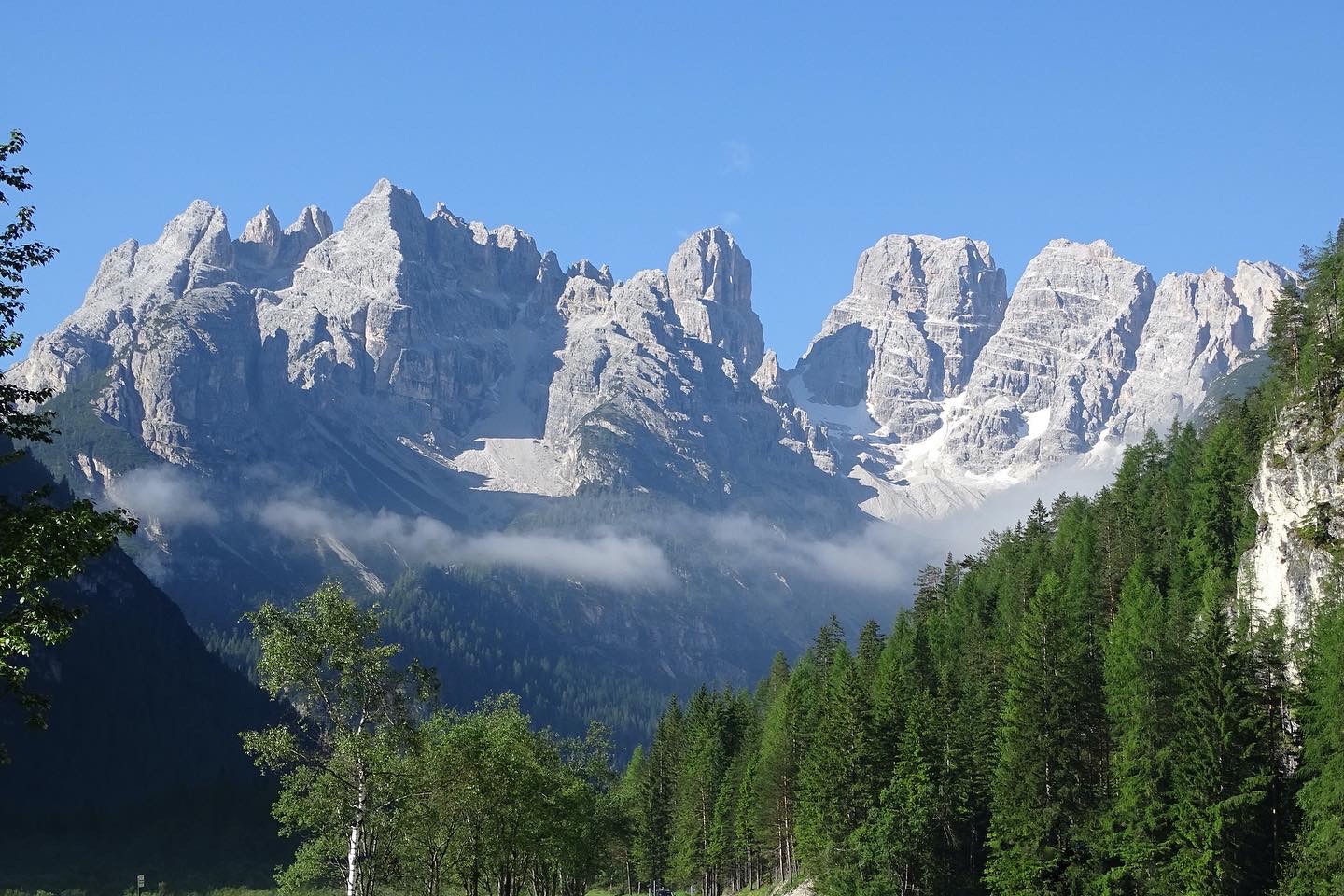
Dolomite is a mineral so common in ancient rocks that it forms mountains like this namesake mountain range in northern Italy. But dolomite is rare in younger rocks and could not be made in the lab under the conditions at which it formed naturally. A new theory helped scientists grow the mineral in the lab at ordinary temperature and pressure for the first time and could help explain the scarcity of dolomite in younger rocks. (Photo: Francesca.z73/Wikimedia Commons)
For 200 years, scientists have failed to grow a common mineral in the laboratory under the conditions believed to have formed it naturally. Now, a team of researchers from the University of Michigan (U-M), USA, and Hokkaido University in Sapporo, Japan have finally pulled it off, thanks to a new theory developed from atomic simulations.
Their success resolves a long-standing geology mystery called the “Dolomite Problem.” Dolomite—a key mineral in the Dolomite mountains in Italy, Niagara Falls, and Utah’s Hoodoos—is very abundant in rocks older than 100 million years, but nearly absent in younger formations.
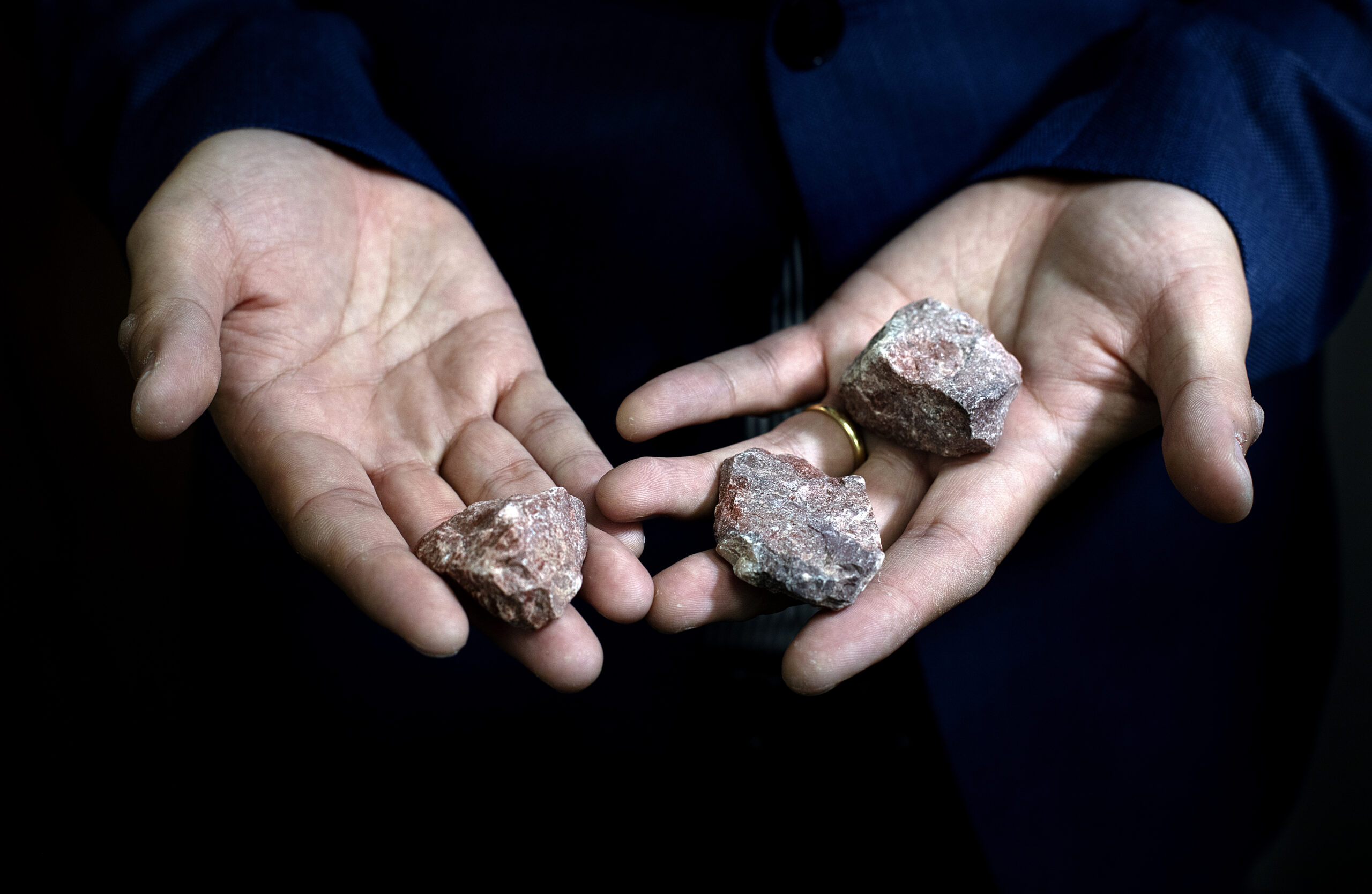
Professor Wenhao Sun shows off dolomite from his personal rock collection. Sun studies crystal growth of minerals from a materials science perspective. By understanding how atoms come together to form natural minerals, he believes we can reveal fundamental mechanisms of crystal growth, which can be used to make functional materials more quickly and efficiently. (Photo: Marcin Szczepanski)
“If we understand how dolomite grows in nature, we might learn new strategies to promote the crystal growth of modern technological materials,” said Wenhao Sun, the Dow Early Career Professor of Materials Science and Engineering at U-M and the corresponding author of the paper published today in Science.
The secret to finally growing dolomite in the lab was removing defects in the mineral structure as it grows. When minerals form in water, atoms usually deposit neatly onto an edge of the growing crystal surface. However, the growth edge of dolomite consists of alternating rows of calcium and magnesium. In water, calcium and magnesium will randomly attach to the growing dolomite crystal, often lodging into the wrong spot and creating defects that prevent additional layers of dolomite from forming. This disorder slows dolomite growth to a crawl, meaning it would take ten million years to make just one layer of ordered dolomite.
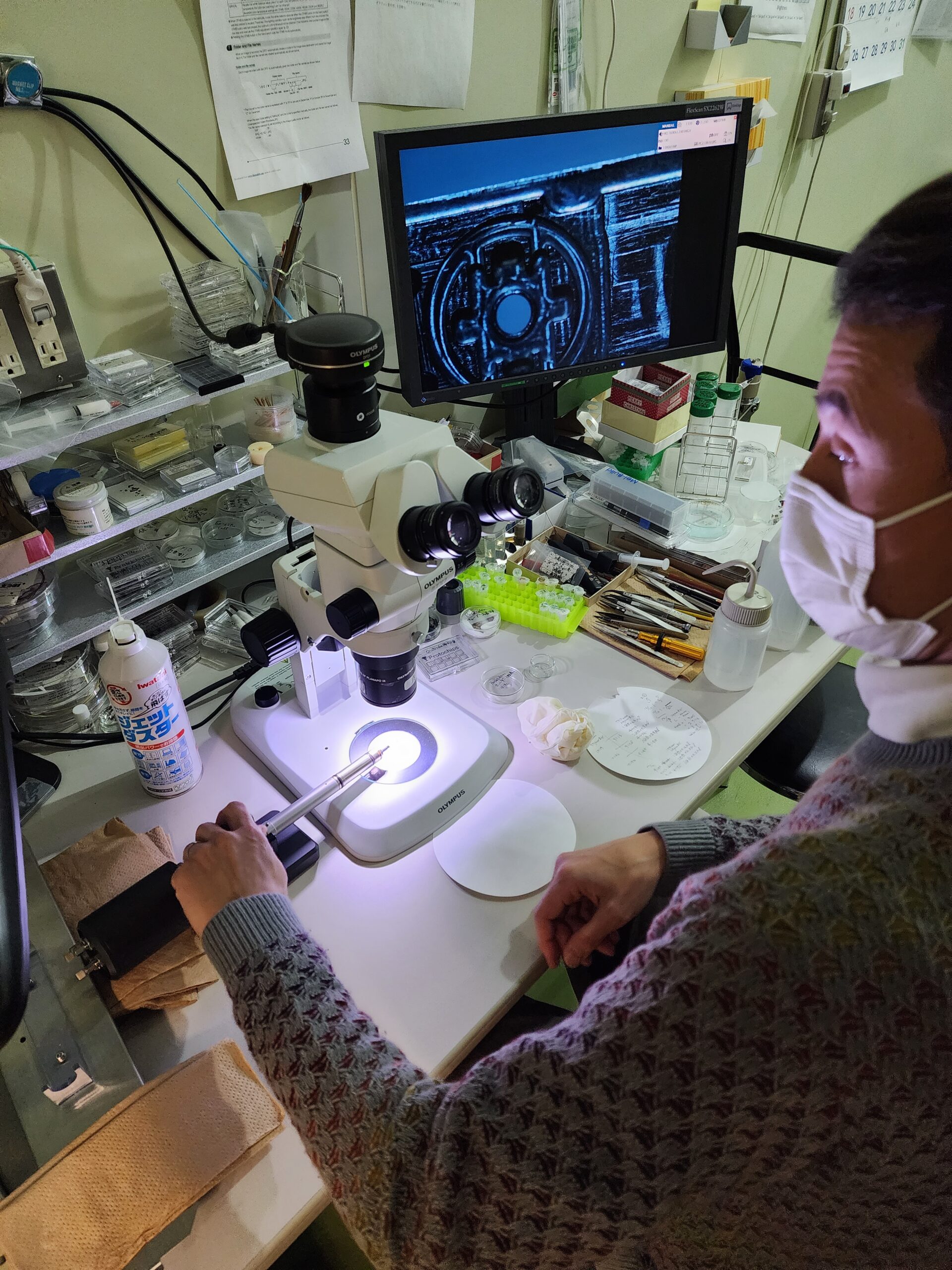
Yuki Kimura, a professor from Hokkaido University in Sapporo, Japan, closely inspects the transmission electron microscope sample holder, which must be free of dust and scratches during the experiment. (Photo credit: Wenhao Sun)
Luckily these defects aren’t locked in place. Because the disordered atoms are less stable than atoms in the correct position, they are the first to dissolve when the mineral is washed with water. Repeatedly rinsing away these defects—for example with rain or tidal cycles—allows a dolomite layer to form in only a matter of years. Over geologic time, mountains of dolomite can accumulate.
To simulate dolomite growth accurately, the researchers needed to calculate how strongly or loosely atoms will attach to an existing dolomite surface. The most accurate simulations require the energy of every single interaction between electrons and atoms in the growing crystal. Such exhaustive calculations usually require huge amounts of computing power, but software developed at U-M’s Predictive Structure Materials Science (PRISMS) Center offered a shortcut.
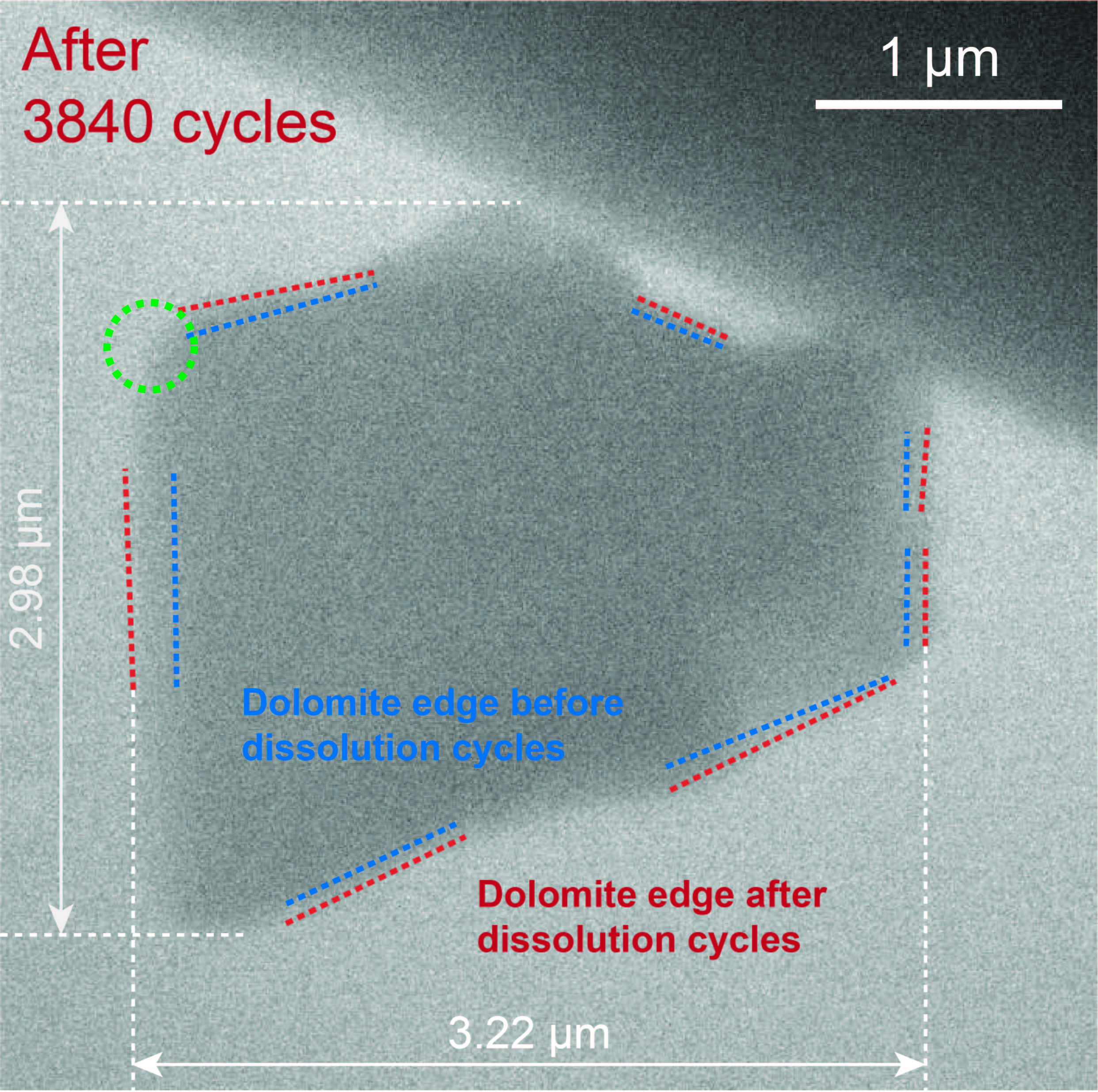
Dolomite is a stable and abundant mineral, but scientists have not been able to grow dolomite since the mineral was first identified in 1791. The research team became the first to explain how dolomite grows in nature, and demonstrated dolomite growth in the lab under a transmission electron microscope. The microscope’s electron beam not only images the tiny crystals, but can also dissolve the top layer, removing defects in the crystal that stop crystal growth. When the beam was turned off, the calcium and magnesium could reattach to the crystal, eventually landing in the ideal arrangements. The blue dashed lines show the crystal boundaries before the experiment, the red lines show the boundaries after the experiment. (Photo: Yuki Kimura)
“Our software calculates the energy for some atomic arrangements, then extrapolates to predict the energies for other arrangements based on the symmetry of the crystal structure,” said Brian Puchala, one of the software’s lead developer and an associate research scientist in U-M’s Department of Materials Science and Engineering. That shortcut made it feasible to simulate dolomite growth over geologic timescales.
“Each atomic step would normally take over 5000 CPU hours on a supercomputer. Now, we can do the same calculation in 2 milliseconds on a desktop,” said Joonsoo Kim, a doctoral student of materials science and engineering and the study’s first author.
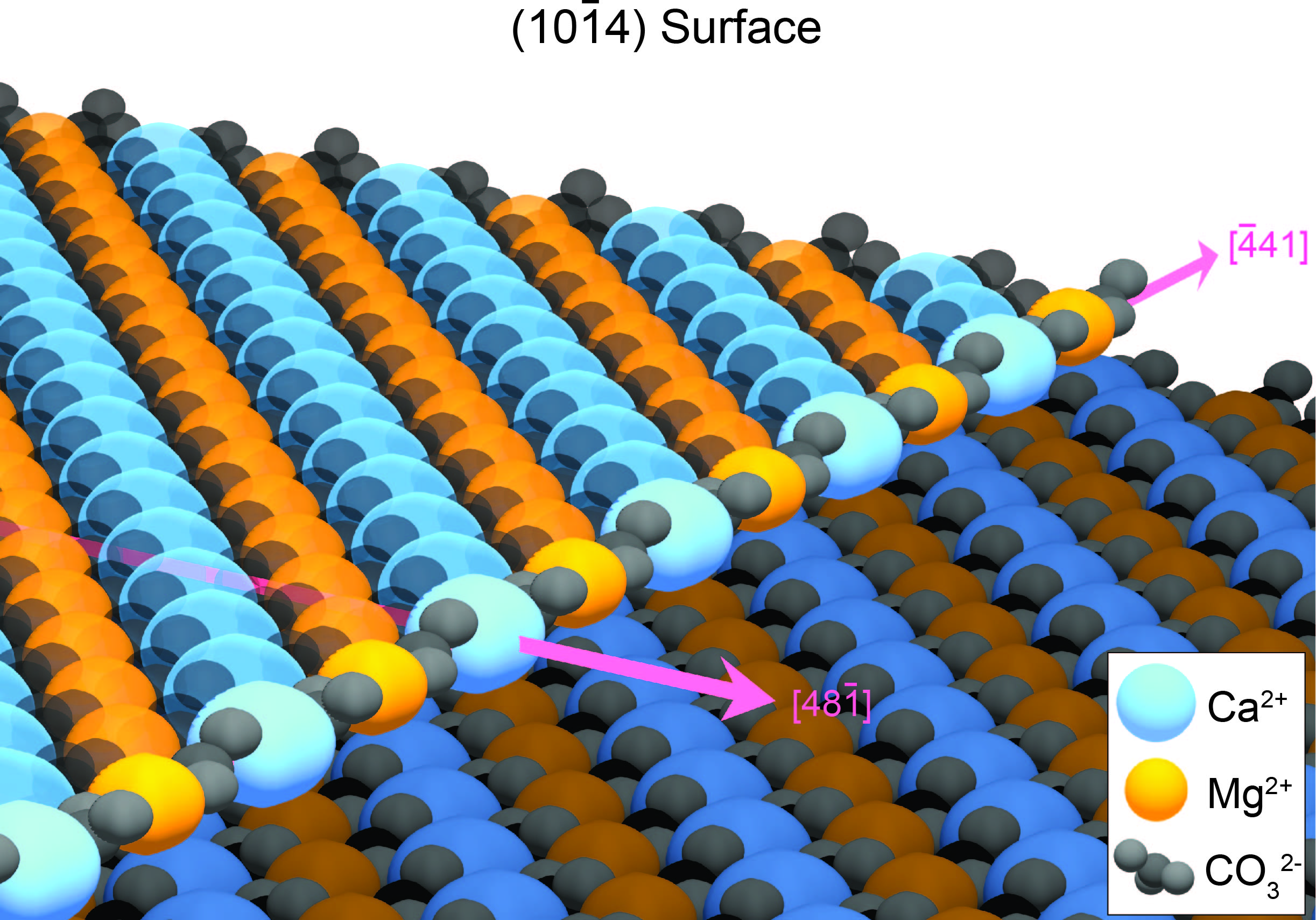
The structure of a dolomite crystal edge. Rows of magnesium (orange spheres) alternate with rows of calcium (blue spheres), and are interspersed with carbonate (black structures). The pink arrows show the directions of crystal growth. Calcium and magnesium often attach to the growth edge improperly, which stops dolomite growth. (Image: Joonsoo Kim)
The few areas where dolomite forms today intermittently flood and later dry out, which aligns well with Sun and Kim’s theory. But such evidence alone wasn’t enough to be fully convincing. Enter Yuki Kimura, a professor of materials science from Hokkaido University, and Tomoya Yamazaki, a postdoctoral researcher in Kimura’s lab. They tested the new theory with a quirk of transmission electron microscopes.
“Electron microscopes usually use electron beams just to image samples,” said Kimura. “However, the beam can also split water, which makes acid that can cause crystals to dissolve. Usually this is bad for imaging, but in this case, dissolution is exactly what we wanted.”
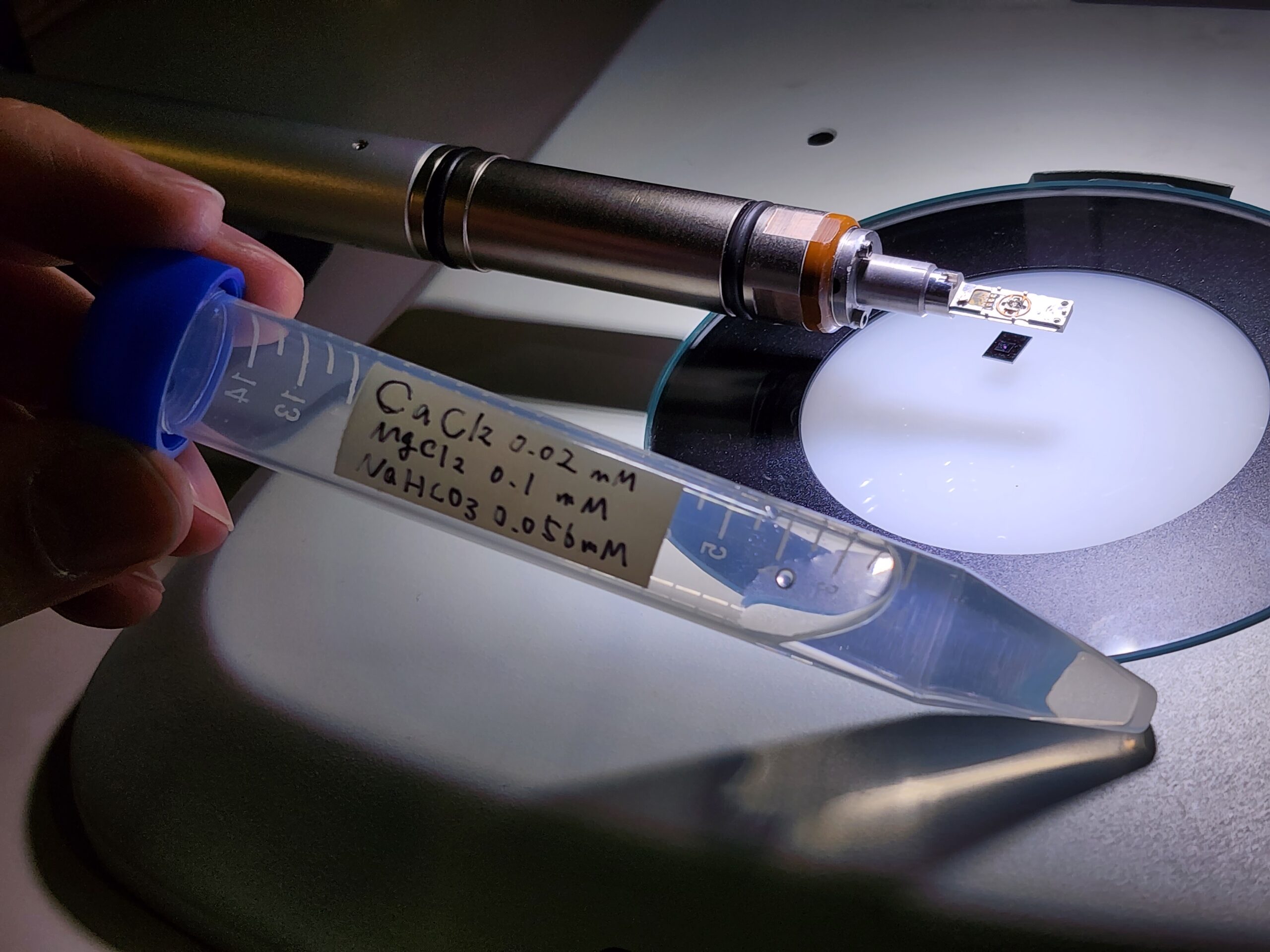
This test tube is holding a concentrated solution of magnesium, calcium, and carbonate ions — the building blocks for a dolomite crystal. The sample holder for the transmission electron microscope behind the test tube will only hold a miniscule amount of this solution — approximately two billionths of a liter. (Photo: Wenhao Sun)
After placing a tiny dolomite crystal in a solution of calcium and magnesium, Kimura and Yamazaki gently pulsed the electron beam 4000 times over two hours, dissolving away the defects. After the pulses, dolomite was seen to grow approximately 100 nanometers—around 250,000 times smaller than an inch. Although this was only 300 layers of dolomite, never had more than 5 layers of dolomite been grown in the lab before.
The lessons learned from the Dolomite Problem can help engineers manufacture higher-quality materials for semiconductors, solar panels, batteries and other tech.
“In the past, crystal growers who wanted to make materials without defects would try to grow them really slowly,” said Sun. “Our theory shows that you can grow defect-free materials quickly, if you periodically dissolve the defects away during growth.”
Original Article:
Joonsoo Kim, et al. Dissolution enables dolomite crystal growth near ambient conditions. Science. November 24, 2023.
DOI: 10.1126/science.adi3690
Funding:
This research was funded by the American Chemical Society Petroleum Research Fund (62081-DNI2), the US Department of Energy (E-SC0012704), and the Japanese Society for the Promotion of Science KAKENHI (20H05657).
Contacts:
Researchers
Assistant Professor Wenhao Sun
Department of Materials Science and Engineering
University of Michigan
Email: whsun[at]umich.edu
Professor Yuki Kimura
Institute of Low Temperature Science
Hokkaido University
Email: ykimura[at]lowtem.hokudai.ac.jp
Institutions
Derek Smith
Communications & Marketing
College of Engineering
University of Michigan
Tel: +1-734-546-3632
Email: smitdere[at]umich.edu
Katherine McAlpine
Communications & Marketing
College of Engineering
University of Michigan
Tel: +1-734-647-7087
Email: kmca[at]umich.edu
Sohail Keegan Pinto (International Public Relations Specialist)
Public Relations & Communications Division
Office of Public Relations and Social Collaboration
Hokkaido University
Tel: +81-11-706-2186
Email: en-press[at]general.hokudai.ac.jp